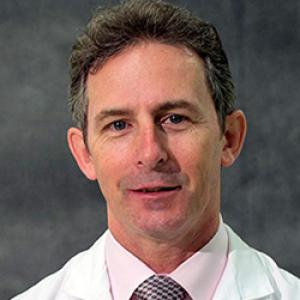
Lab Description
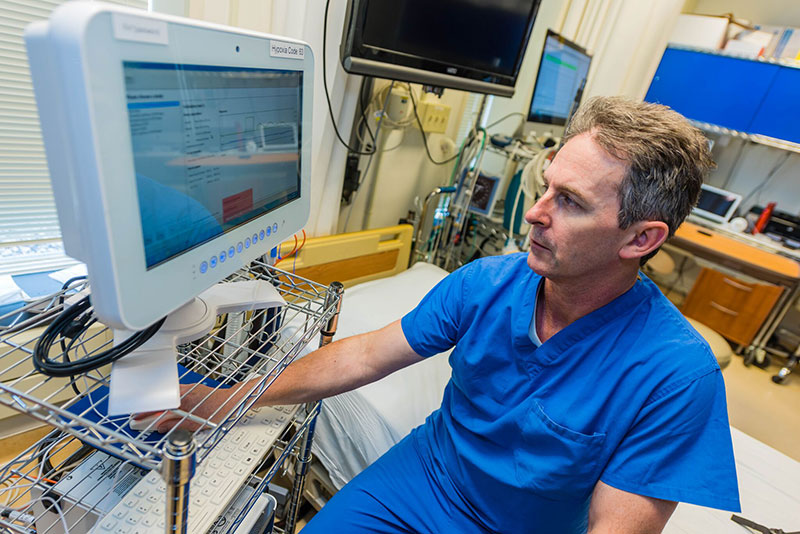
The Human Pharmacology and Physiology Lab is a continuation of the Human Pharmacology Lab originally founded by Professor Peter Glass. The lab is now co-located within the Duke Early Phase Clinical Research Unit (DEPCRU) in Duke Clinics. Studies are typically conducted as a single subject, but it is possible to expand data collection up to four subjects at a time.
The lab is staffed by anesthesiologists, who perform their clinical duties in the operating room (OR). The OR can be a challenging environment to perform research in order to assess the effect of a new anesthetic agent or the accuracy of a new monitor. The conduct of research in healthy volunteers within the HPPL allows specific measurement of multiple physiological parameters in response to key physiological perturbations in the absence of potential confounders. A prime example was the evaluation of a new pulse oximeter-based device to assess the extent of hypovolemia (blood loss) with up to 25 percent total blood volume removed under controlled conditions. This would not have been possible in surgical patients under anesthesia.
Major Research Interests
Cerebral Oximeter Studies
The use of near infra-red spectroscopy (NIRS) to estimate non-invasively the oxygen saturation of brain tissue was first described in 1977 by the Duke biomedical engineer, Franz Jobsis. The principles of spectroscopy, whether NIRS (700 – 900 nm) or visible light used in the familiar finger pulse oximeter, have been well described. When a known amount of light is emitted from a sensor there is a decrement in the amount of light reaching the sensor detector - this is termed attenuation. Of the total amount of attenuation, 80% can be attributed to scattering of light photons within the tissue and 20% to absorption by biological molecules, of which hemoglobin is an example. Oximeters measure the amount of light attenuation as the light passes through the tissue bed. Based upon the assumption that the light scattering will remain constant, any changes in light attenuation will be attributed to changes in the amount of light absorption by the biological tissue. This establishes a link between light absorption and the biological tissue, which is then tested over a clinically relevant range. First a change in the condition of the biological tissue is created and then the subsequent change in the amount of light attenuation/absorption is measured. A good example is arterial blood oxygen saturation and pulse oximeters. A series of arterial blood samples are taken at different levels of oxygen saturation between 70 – 100% and the amount of light absorbed at each level is recorded by the pulse oximeter. The exact value of arterial oxygen saturation (SaO2) is measured independently by a blood gas machine termed a co-oximeter. A correlation is then established between the biological variable, SaO2, and the amount of light absorbed, which is then interpreted and displayed as a non-invasive estimate of the arterial oxygen saturation by the pulse oximeter (SpO2).
Cerebral oximeters share many similarities with pulse oximeters but there are a couple of distinct, but important, differences. Visible light, as used in pulse oximeters, has a limited depth of tissue penetration (~ 1 cm) and cannot pass through bone. In contrast, NIRS can pass through the bone of the skull to a depth of several centimeters making it suitable for cerebral oximetry to estimate the oxygen level in brain tissue. Another difference is that pulse oximetry is only interrogating the arterial blood of the tissue whereas cerebral oximetry estimates both arterial and venous components, of which the venous predominates in terms of blood volume within the brain. The measured light attenuation (and, by interpolation as described above, absorption) must be correlated with a biological variable. In the absence of the ability to directly sample the blood within the brain tissue (analogous to arterial blood for pulse oximeters) a surrogate value must be established. The saturation value of the surrogate must lie between the anatomical sites of cerebral blood input (carotid artery) & outflow (jugular vein). The surrogate saturation value is a composite of a weighted equation of co-oximeter measured oxygen saturations from arterial/venous blood samples. In a similar manner to the development and testing of pulse oximeters, the correlation between the displayed oximeter saturation derived from the amount of light absorbed and the biological variable is established.
The FDA has published the Guidance for Industry and FDA Staff - Pulse Oximeters - Premarket Notification Submissions [510(k)s]. This document provides guidance for manufacturers in the preparation of a premarket notification submission. In particular there is a specific recommendation (Section 4.1.1) on how oximeter and blood gas data from healthy volunteers should be obtained – referenced to the publication ISO 80601-2-61:2011 Annex EE.2 Procedure for invasive laboratory testing on healthy volunteers. The FDA has not published the equivalent Guidance document for cerebral oximeters, though manufacturers of cerebral oximeter are still required to submit information to obtain premarket notification approval. In the absence of a formal Guidance manufacturers have elected to use the recommendations detailed in the pulse oximeter Guidance. To date this practice has been accepted by the FDA as appropriate. There are several additional methods pertinent to cerebral oximeters studies that have been incorporated into study protocols during previous 510(k) applications and have established the basis for subsequent applications: the placement of a jugular bulb catheter, the rate of jugular bulb sampling, the control of arterial carbon dioxide (normocarbia) through alteration of inspired gas mixtures and the use of a weighted arterial:venous equation to estimate cerebral tissue oxygen saturation. The first cerebral oximeter was introduced into clinical practice in 1996. Thereafter other manufacturers have introduced additional, more sophisticated cerebral oximeters as clinical use, predominantly in anesthesiology, has broadened. The HPPL has successfully completed over 350 desaturation runs in healthy volunteers without any serious adverse events.
Hypovolemia
The Advanced Trauma Life Support (ATLS) course defines four classes of hemorrhagic shock, based upon the percentage of estimated blood loss: 0 – 15% (Class 1); 15 – 30% (Class 2); 30-40% (Class 3) & > 40% (Class 4). The clinical features are often mild or subtle in Classes 1 & 2, especially in young, healthy individuals. Tachycardia and decrease in blood pressure often do not manifest until Class 3. The early recognition of ongoing shock is therefore clinically challenging. The development of the Compensatory Reserve Index (CRI), based upon the pulse waveform measured by a pulse oximeter, provides a new method of providing vital information to the clinician in the assessment of volume status. Two studies were completed by the HPPL: CRI 1 in which 20% total blood volume was removed via peripheral IV catheter and CR1 2 in which 25% total blood volume was removed via central line catheter.
Carbon Monoxide Pulse Oximeter
The pulse oximeter oxygen saturation (SpO2) is a non-invasive estimate of the arterial blood oxygen saturation (denoted as SaO2). Hemoglobin is the molecule in red blood cells to which oxygen binds. The binding and subsequent unbinding produces oxyhemoglobin [HbO2] and deoxyhemoglobin [Hb], respectively. Hemoglobin may also bind to other substances, such as carbon monoxide [COHb] or methemoglobin [MetHb], which eliminate the oxygen-carrying capability of hemoglobin and render it as non-functional (dysfunctional). The SaO2 represents the fraction (%) of HbO2in relation to the total hemoglobin [all types = HbO2 + Hb + COHb + MetHb]. This is termed fractional SaO2. If no dysfunctional hemoglobins are present, then the equation for total hemoglobin can be modified with the removal of COHb & MetHb [total hemoglobin = HbO2 + Hb]. This is termed functional SaO2. Under normal circumstances, fractional and functional SaO2 will be equivalent. Typical pulse oximeters are unable to measure either COHb or MetHb and so SpO2 is an estimate of the functional SaO2.
However, in the presence of dysfunctional hemoglobins is present, fractional and functional SaO2 will no longer be equal. For example, when the COHb is 10% in heavy smokers, the fractional SaO2 will be 90% in contrast to functional SaO2 of 100%. More importantly, the pulse oximeter will report SpO2 of 100%. The ability of pulse oximeters to correctly report the functional SaO2 has strong clinical merit, such as carbon monoxide poisoning.
In this study the accuracy of a new pulse oximeter to estimate COHb up to 15% at different levels of SaO2 down to 85% was measured.
Phase 1 Drug Studies
These are pharmacokinetic studies where the study drug is administered at a specific time (T=0) followed by frequent blood sample draws at known time points. Subsequently the plasma concentrations of the study drug can be plotted against time from which information about drug disposition (such as maximum plasma concentration (Cmax), time to Cmax, elimination half-life) can be calculated. This also provides information to predict how future drug administration (different dose, bolus versus infusion) may behave. Pharmacodynamic measures can also be completed, such as depth of sedation and analgesia, to determine how plasma concentration correlates with the anticipated clinical effect.
High Altitude
In conjunction with exercise physiologists from University of British Columbia, Okanagan (UBCO), Canada HPPL members have participated in research studies at the Pyramid Lab, close to Everest base Camp. The focus has been on the effect of ascent to high altitude upon cerebral blood flow.
Apnea Divers & Breath Hold Tolerance
In conjunction with exercise physiologists from UBCO and University of Split, Croatia HPPL members have participated in research studies of elite apnea (breath hold divers). The focus has been to determine the underlying physiological mechanism responsible for terminating a breath hold.
Study Participation
The process to participate in a study is relatively straightforward. Following Duke IRB approval, potential study participants can read about the study via a study-dedicated REDCap website with the informed consent form. Subjects will then complete an online REDCap survey in which questions about the major inclusion/exclusion criteria are sought. Once the HPPL Clinical Research Coordinator (CRC) has reviewed the survey, eligible subjects will be contacted to arrange a date/time for a screening visit. At the screening visit, the principal investigator (PI) will discuss in person the details of the study. Specific topics will include the study objectives, study participant involvement, details of line placement/drug administration/gas mixture administration, potential complications and risks and the informed consent process. If subjects wish to proceed they will then sign the informed consent form (ICF). A full medical history and physical examination will then be completed. Study-specific investigations such as ECG, lung function test and blood samples will be performed as appropriate. The PI will make the final decision of study eligibility once all of the information is available. Eligible study participants will then be asked to attend the HPPL on a separate day to complete the Study Visit. Most studies are completed with a single study day visit of four hours with discharge to home. Some studies may require overnight admission to DEPCRU or repeated visits.
Study Equipment
Data Acquisition
The ccNexfin is a capable of non-invasive, continuous measurement of blood pressure. The additional parameters of cardiac output (CO), stroke volume (SV) and systemic vascular resistance (SVR) are derived. The ExSpiron respiratory monitor uses a single pad placed upon the thoracic wall to measure non-invasively tidal volume, respiratory rate and minute volume. The Oridion Capnostream monitor is capable of measuring end-tidal capnography (ET CO2), respiratory rate, pulse oximetry (SpO2) and pulse rate. The addition of ADInstruments ECG bioamp [FE312] provides a comprehensive non-invasive data collection system of cardiovascular and respiratory parameters. The further addition of ADInstruments BP amp [FE117] pressure transducers can be attached to invasive catheters to measure arterial, central venous or jugular bulb pressures. The monitors are mounted onto a mobile cart which allows analog output from the ccNexfin, Oridion Capnostream and ADInstruments bioamps to be coordinated through the data capture platform, ADInstruments PowerLab. This is in turn linked to the ADInstruments LabChart software program. The input signals from the different monitors are automatically time synchronized. Waveforms, such as the ECG and arterial pressure, are captured at a rate of 1 kHz. The use of pre-populated comments allows the of time-sensitive events e.g. blood sample, start/stop of drug infusion. The real-time display of the various parameters can configured, dependent upon the needs of the study. Once the study is completed, LabChart permits the export of data in a variety of formats for further analysis, such as .txt or MatLab. The lab has four (4) monitoring towers which can be deployed to adjacent rooms within the DEPCRU when warranted by the study. The extensive data collection during a study provides two benefits: (1) reporting of multiple parameters at serial time points e.g. heart rate, SpO2, BP at 5-minute intervals and (2) additional useful insight in the event of an unexpected event e.g. hypotension due to drug-induced vasodilation demonstrated by reduced systemic vascular resistance followed by a drop in cardiac output.
Gas Delivery Systems
Controlled delivery of precise gas concentrations of oxygen and/or carbon dioxide are used in differing protocols. The RA-MR, manufactured by Thornhill Research, is a dedicated gas blender capable of achieving specific end-tidal O2 and CO2 concentrations, independent of the subject’s minute ventilation. For cerebral oximeter validation it is necessary to reduce the SpO2 in 5% increments from 100 to 70% (ET O2 38 mmHg) with maintenance of ET CO2 at 40 mmHg (isocapnia). The Environics ROBD2, originally designed by US Navy for use in jet fighter simulators, is a gas blender capable of reducing the inspired oxygen concentration from 21% (room air) to 4.4% (equivalent to altitude of 32,000 feet).
Quantitative Sensory Testing (QST) & Pain Testing
- Neurotron Neurometer CPT: electrical-based device
- Medoc TSA-II: thermal-based device
- Medoc Algometer: pressure-based device
Blood Sampling
- Arterial and venous blood samples can easily be drawn for PK analysis. The DEPCRU lab has appropriate centrifuges and freezers to process blood samples in accordance with PK sample processing instructions.
- The HPPL has two (2) Radiometer ABL90 FLEX blood gas analyzers onsite within the lab for immediate blood gas sample analysis.
Additional Equipment
- Mortara ECG telemetry is available throughout the DEPCRU permitting continuous 12-lead ECG data collection.
- Sonosite NanoMaxx portable ultrasound machine to assist with arterial and central venous catheter placement.
- BIS Bilateral Sensor and Masimo Sedline monitors for continuous level of consciousness monitoring.
- Neuroptics PL200 pupillometer for measurement of pupil size.
Selected Publications
Cerebral Oximeter Studies
MacLeod DB, Ikeda K, Vacchiano C, Lobbestael A, Wahr JA, Shaw AD. Development and Validation of a Cerebral Oximeter Capable of Absolute Accuracy. J. Cardiothorac. Vasc. Anesth. 2012;26(6):1007–14.
Ikeda K, MacLeod DB, Grocott H, Moretti E, Ames W, Vacchiano C. Accuracy of NIRS cerebral oximetry device & its potential value for estimating jugular venous oximetry. Anesth Analg 2014; 119(6):1381-92.
Benni PB, MacLeod DB, Ikeda K, Lin H-M. A validation method for near-infrared spectroscopy based tissue oximeters for cerebral and somatic tissue oxygen saturation measurements. J Clin Monit Comput. 2017;22(5):596–16. doi:10.1007/s10877-017-0015-1.
Pulse Oximeters
MacLeod DB, Cortinez LI, Keifer JC, Cameron D, Wright DR, White WD, Moretti EW, Radulescu LR, Somma J. The desaturation response time of finger pulse oximeters during mild hypothermia. Anaesthesia 2005: 60:65-70.
Dubowitz G, Breyer K, Lipnick M, Sall JW, Feiner J, Ikeda K, MacLeod DB and Bickler PE. Accuracy of the Lifebox pulse oximeter during hypoxia in healthy volunteers. Anaesthesia 2013; 68(12):1220-3.
Bickler P, Feiner J, Lipnick M, Batchelder P, MacLeod DB, Severinghaus J. Effects of acute, profound hypoxia on healthy humans: Implications for safety of tests evaluating pulse oximetry or tissue oximetry performance. Anesthesia & Analgesia. 2016;124(1):146-153.
Hypovolemia
Convertinto VA, Howard JT, Hinojosa-Laborde C, Cardin S, Batchelder P, Mulligan J, Grudic GZ, MacLeod DB. Individual-specific, beat-to-beat trending of significant human blood loss: the compensatory reserve. Shock. 2015;44 Suppl 1:27-32. doi:10.1097/SHK.0000000000000323
Moulton SL, Mulligan J, Santoro MA, Bui K, Grudic GZ, MacLeod DB. Validation of a Non-invasive Monitor to Continuously Trend Individual Responses to Hypovolemia. Accepted for publication by Journal of Trauma and Acute Care Surgery. 2017: doi:10.1097/TA.0000000000001511
Phase 1 Drug Studies
Hsu Y, Cortinez LI, Robertson KM, Keifer JC, Sum-Ping ST, Moretti EW, Young CC, Wright D, MacLeod DB, Somma J. Dexmedetomidine pharmacodynamics, part I: crossover comparison of the respiratory effects of dexmedetomidine and remifentanil in healthy volunteers. Anesthesiology 2004; 101(5): 1066-76.
Hsu Y, Cortinez LI, Robertson KM, Keifer JC, Sum-Ping ST, Moretti EW, Young CC, Wright D, MacLeod DB, Somma J. Dexmedetomidine pharmacodynamics, part II: crossover comparison of the analgesic effect of dexmedetomidine and remifentanil in healthy volunteers. Anesthesiology 2004; 101(5): 1077–83.
MacLeod DB, Habib AS, Ikeda K, Spyker DA, Cassella JV, Ho KY, et al. Inhaled fentanyl aerosol in healthy volunteers: pharmacokinetics and pharmacodynamics. Anesth. Analg. 2012 Nov;115(5):1071–7.
High Altitude
Willie CK, Smith KJ, Day TA, Ray LA, Lewis, NCS, Bakker A, MacLeod DB, Ainslie PN. Regional cerebral blood flow in humans at high altitude: gradual ascent and two weeks at 5050m. J Applied Physiology 2014; 116:905-10.
Apnea Divers & Breath Hold Tolerance
Willie CK, Ainslie PN, Drvis I, MacLeod DB, Bain A, Madden D, Zubin Maslov P, Dujic Z. Regulation of blood brain flow & oxygen delivery in elite breath-hold divers. J Cerebral Blood Flow & Metab 2015; 35:66-73.
Bain A, Ainslie P, Hoiland R, Willie, CK, MacLeod DB, Madden D, Maslov P, Drvis I, Dujic Z. Role of cerebral blood flow in extreme breath holding. Translational Neuroscience. 2016;7(1):12-16.
Bailey DM, Willie CK, Hoiland RL, Bain AR, Macleod DB, Santoro MA, DeMasi DK, Andrijanic A, Mijacika T, Barak OF, Dujic Z, Ainslie PN. Surviving Without Oxygen: How Low Can the Human Brain Go? High Alt Med Biol. 2017;18(1):73-79.